Steffen-Sebastian Bolz
MD, PhD
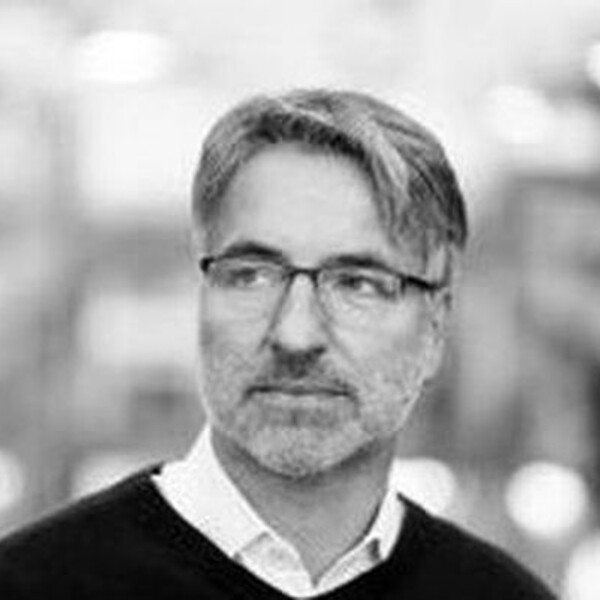
Qualification
- MD, PhD
Professional Memberships
- Heart & Stroke/Richard Lewar Centre of Excellence for Cardiovascular Research, La Ka Shing Knowledge Institute/ Keenan Research Centre, St. Michael's Hospital
Director, Toronto Centre for Microvascular Medicine
Research Synopsis
Research Interests: Signaling pathways that calcium-independently regulate resistance artery tone.
Keywords: Resistance arteries, Ca2+-independent vasoconstriction, myogenic vasoconstriction, blood flow regulation, sphingosine-1-phosphate signaling, TNFalpha signaling, cystic fibrosis transmembrane regulator (CFTR), bioimaging, protein trafficking, transfection.
Detailed Description: Resistance arteries are highly specialized structures. Their circularly-orientated smooth muscle cells allow for rapid changes in vessel diameter and thus, alterations in microvascular resistance and capillary perfusion. The intrinsic property of resistance arteries to antagonize an abrupt elevation in systemic blood pressure via an increase in vascular resistance (i.e., the myogenic response or Bayliss effect) plays an important role in the autoregulation of blood flow. In addition, the interplay between elevations in blood pressure (e.g., caused by augmented cardiac output) and the subsequent pressure-induced increases in peripheral resistance establishes a positive feedback mechanism, which can prominently contribute to an elevation in systemic blood pressure (hypertension). Despite its obvious importance in physiological and pathophysiological processes, our knowledge of resistance artery physiology is relatively limited.
Our laboratory group has invested substantial effort to develop the isolated resistance artery model into a versatile experimental platform. A major breakthrough that formed the basis for many later developments was the establishment of a culture protocol that allows for maintenance of the structural and functional integrity of isolated arteries for up to five days. This was later complemented by the evolution of a protocol that allows for highly efficient transfection of resistance artery wall cells. For the first time, this innovative protocol allowed targeted alteration of the genetic background of an isolated resistance artery with a highly efficient non-viral method. The inherent power is its independence from the availability of chemical inhibitors to manipulate signaling pathways of interest. Microvascular research can now fully benefit from the advanced knowledge provided by the different species-specific genome projects and pursue state of the art molecular approaches.
We have employed this technology (i) to characterize the mode of action of the endothelium-derived hyperpolarizing factor in resistance arteries and to identify its chemical nature (ii) to investigate mechanisms of oxLDL-induced vasoconstriction in resistance arteries and (iii) to demonstrate that NO-induced activation of the myosin light chain phosphatase in microvascular smooth muscle cells antagonizes proconstrictory Ca2+-independent pathways that terminate in inhibiting this enzyme. In summary, these results obtained by using our new platform technology contributed to an advanced understanding of microvascular tone regulation and supported the innovative concept of Ca2+-independent tone regulating mechanisms in the microcirculation.Based on these results, our group has searched for relevant upstream modulators of the RhoA/Rho kinase signaling pathway and identified endogenous sphingosine-1-phosphate (S1P) generated by sphingosine kinase as one of the major determinants of the myogenic response. We proposed a new concept in this study that was the first to concisely explain the regulation and orchestration of the initial Ca2+-dependent and the subsequent Ca2+-independent phase of this important vascular response.
In this context, we have elucidated (i) the molecular mechanisms underlying the pressure-induced activation of smooth muscle (myogenic) S1P signaling in resistance arteries, (ii) their relevance in maintaining increased peripheral resistance during heart failure and (iii) their role in several disease states (i.e., heart failure, subarachnoid haemorrhage, hearing loss and diabetes). More recently, our research has characterized a mandatory role for the Cystic Fibrosis Transmembrane Conductance Regulator (CFTR) in the S1P degradation pathway and imbued CFTR with a key role in the regulation of microvascular tone. The key function of smooth muscle CFTR is to import extracellular S1P to the intracellular compartment prior to its hydrolyzation by S1P phosphohydrolase, an intracellular enzyme residing in the sER membrane. Through our translational work with several disease models, the TNFa-dependent regulation of CFTR expression and membrane presence emerged as a primary principle regulating microvascular S1P bioavailability. The inhibition of CFTR function by TNFa (through down regulation of its expression and its sequestration from the plasma membrane) is the basis for increased myogenic responsiveness in a variety of microvascular beds in heart failure, subarachnoid haemorrhage, and diabetes. Consequently, our research has validated the anti-TNFa drug etanercept (Enbrel) as an effective treatment to correct cerebral perfusion deficits as a common complication of heart failure.
Following our translational strategy to ultimately provide an experimental platform that will allow personalization of microvascular medicine, we (in collaboration with Dr. Axel Guenther, UofT) have recently developed a proprietary platform, the Artery-on-a-Chip technology (AoC), which overcomes the limitations of classical pressure myography and completely redefines the experimental approach. The AoC facilitates resistance artery handling/assessment, reduces cost and time per experiment by several orders of magnitude and enables fully automated experiments. All essential procedures are integrated onto a polymer-based chip, where small arteries can be studied in a near in vivo microenvironment. The AoC will allow us to (a) translate rodent model data to human arteries and (b) promote the development of the AoC platform into a diagnostic tool for on site assessment of a patient’s microvascular status.
METHODS USED
Cell and tissue culture: Artery cultures, brain slice, endothelial cells, smooth muscle cells.
Procedures: Artery on a chip method, behavioural tests, isolated vessel preparation, mass spectrometry, qRT-PCR, RIA, RT-PCR, signal transduction characterization, siRNA, vessel cannulation, western blot.
EQUIPMENT USED
Calcium imaging system (PTI), deconvolution fluorescence microscope (ZEISS Marianas system), dissecting microscope (Leica MZ16).
PRESENT TRAINEES
Jeffrey Kroetsch
Meghan Sauve
Sonya Hui
Firhan Malik
Darcy Lidington
Andrew Levy
PRESENT COLLABORATIONS
Within the Department of Physiology:
Gregory Hare
Peter Backx
Patricia Brubaker
Stephen Matthews
Mansoor Husain
Scott Heximer
Wolfgang Kuebler
Outside the Department of Physiology:
Stefan Offermanns, Max Planck Institute for Heart and Lung Research Bad Nauheim, Germany
Avril Somlyo, University of Virginia, Charlottesville, USA
Sarah Spiegel, University of Virginia, Richmond, USA
Rudolf Schubert, University of Heidelberg, Campus Mannheim, Mannheim, Germany
Loch MacDonald, St. Michael’s Hospital, Toronto, Canada
Recent Publications
Stroke. 2015 Jul 2. pii: STROKEAHA.114.006365. [Epub ahead of print]
Therapeutically Targeting Tumor Necrosis Factor-α/Sphingosine-1-Phosphate Signaling Corrects Myogenic Reactivity in Subarachnoid Hemorrhage.
Courses
Course Number: PSL 304, PSL372, PSL1080, PSL462, MSC1010, JCV3063, STF1010Course Name: